In a bustling town, four curious friends—Atom, Molecule, Solid, and Gas—decided to explore the world of particles. Atom, the smallest, whispered secrets of existence, while Molecule danced gracefully, forming bonds with others. Solid stood firm, showcasing strength and structure, while Gas floated freely, spreading joy with its lightness. Together, they revealed the four particle theory: everything is made of atoms, which combine to form molecules, and these can exist as solids, liquids, or gases. Their adventure taught the town that understanding the unseen could unlock the mysteries of the universe.
Table of Contents
- Exploring the Foundations of Particle Theory
- Understanding the Four Fundamental Forces
- Applications of Particle Theory in Modern Science
- Future Directions and Innovations in Particle Research
- Q&A
Exploring the Foundations of Particle Theory
At the heart of modern physics lies a framework that seeks to explain the fundamental constituents of matter and the forces that govern their interactions. This framework, often referred to as particle theory, posits that everything in the universe is composed of tiny, discrete particles. These particles can be categorized into various types, each with unique properties and behaviors. Understanding these categories is essential for grasping the complexities of the universe.
One of the primary categories within particle theory is **fermions**, which include particles such as electrons, protons, and neutrons. Fermions are characterized by their adherence to the Pauli exclusion principle, which states that no two identical fermions can occupy the same quantum state simultaneously. This property is crucial for the formation of matter, as it explains the structure of atoms and the stability of the elements that make up our world. Fermions are further divided into **leptons** and **quarks**, each playing a vital role in the composition of matter.
In contrast to fermions, we have **bosons**, which are force-carrying particles that mediate interactions between fermions. Bosons include well-known particles such as photons, gluons, and the Higgs boson. Unlike fermions, bosons do not follow the Pauli exclusion principle, allowing multiple bosons to occupy the same quantum state. This unique characteristic enables them to facilitate the fundamental forces of nature, including electromagnetism, the strong nuclear force, and the weak nuclear force, thereby shaping the interactions that govern the universe.
Lastly, the concept of **antimatter** introduces a fascinating dimension to particle theory. For every particle, there exists a corresponding antiparticle with the same mass but opposite charge. When particles and antiparticles meet, they annihilate each other, releasing energy in the process. This phenomenon not only highlights the symmetry in the universe but also raises intriguing questions about the nature of matter and the potential for antimatter to play a role in future technologies, such as advanced propulsion systems or energy sources. The exploration of these foundational elements continues to inspire physicists and researchers as they delve deeper into the mysteries of the cosmos.
Understanding the Four Fundamental Forces
The universe is governed by four fundamental forces that dictate the interactions between particles and the structure of matter itself. These forces are the **gravitational force**, **electromagnetic force**, **strong nuclear force**, and **weak nuclear force**. Each of these forces plays a crucial role in shaping the cosmos, from the smallest subatomic particles to the grandest galaxies.
The **gravitational force** is perhaps the most familiar of the four. It is the force of attraction that pulls objects toward one another, influencing everything from the falling of an apple to the Earth to the orbits of planets around the sun. This force is relatively weak compared to the others, yet it has an infinite range and is responsible for the large-scale structure of the universe, including the formation of stars, planets, and galaxies.
Next is the **electromagnetic force**, which governs the interactions between charged particles. This force is responsible for electricity, magnetism, and light, making it essential for the existence of atoms and molecules. It is significantly stronger than gravity and operates over infinite distances, but it can be shielded or canceled out by other charges. The electromagnetic force is what holds electrons in orbit around the nucleus of an atom, enabling the formation of chemical bonds and the diversity of matter.
The **strong nuclear force** is the powerhouse that binds protons and neutrons together in atomic nuclei. It is the strongest of the four forces, but it operates over a very short range, typically only a few femtometers. This force is crucial for the stability of atoms, as it overcomes the electromagnetic repulsion between positively charged protons. Without the strong nuclear force, atomic nuclei would not exist, and the universe as we know it would be drastically different.
the **weak nuclear force** is responsible for processes such as beta decay, where a neutron transforms into a proton, emitting an electron and an antineutrino. This force is essential for the nuclear reactions that power stars, including our sun. Although it is weaker than both the strong nuclear force and electromagnetic force, it plays a vital role in the processes that govern the life cycles of stars and the synthesis of elements in the universe.
Applications of Particle Theory in Modern Science
Particle theory serves as a foundational concept in various fields of modern science, influencing our understanding of matter and its interactions. In chemistry, for instance, the behavior of gases can be explained through the kinetic molecular theory, which posits that gas particles are in constant motion. This theory helps scientists predict how gases will react under different conditions, such as changes in temperature and pressure. By applying particle theory, chemists can design more efficient reactions and develop new materials with specific properties.
In the realm of physics, particle theory is crucial for understanding the fundamental forces of nature. The Standard Model of particle physics describes how particles like quarks and leptons interact through fundamental forces mediated by bosons. This framework not only explains the behavior of subatomic particles but also provides insights into the origins of the universe. Researchers utilize particle accelerators to explore these interactions, leading to groundbreaking discoveries such as the Higgs boson, which confirms the existence of mass in particles.
Biology also benefits from particle theory, particularly in the study of cellular processes. The movement of molecules across cell membranes is governed by principles derived from particle theory, such as diffusion and osmosis. Understanding these processes is essential for fields like pharmacology, where the effectiveness of drug delivery systems relies on how particles interact with biological membranes. By leveraging particle theory, scientists can enhance drug formulations and improve therapeutic outcomes.
Moreover, advancements in nanotechnology are deeply rooted in particle theory. At the nanoscale, materials exhibit unique properties that differ significantly from their bulk counterparts. By manipulating particles at this scale, researchers can create innovative materials with tailored characteristics, such as increased strength or enhanced conductivity. This application of particle theory is paving the way for breakthroughs in electronics, medicine, and energy storage, showcasing the versatility and importance of understanding particles in modern science.
Future Directions and Innovations in Particle Research
As we look to the horizon of particle research, the integration of advanced technologies is poised to revolutionize our understanding of fundamental particles. Innovations in quantum computing are expected to enhance simulations and modeling of particle interactions, allowing researchers to explore complex phenomena that were previously beyond reach. This leap in computational power will enable scientists to test theoretical predictions with unprecedented accuracy, potentially leading to new discoveries in particle physics.
Moreover, the development of next-generation particle accelerators promises to push the boundaries of our current knowledge. These facilities will not only increase collision energies but also improve detection capabilities, enabling the observation of rare particle events. With the potential to uncover new particles or interactions, these advancements could provide critical insights into the fundamental forces that govern the universe.
Collaboration across disciplines is also becoming increasingly vital in particle research. By merging insights from astrophysics, materials science, and computational biology, researchers can approach particle theory from multiple angles. This interdisciplinary approach may lead to innovative methodologies and experimental designs, fostering a more holistic understanding of particle behavior and its implications for the cosmos.
the rise of machine learning and artificial intelligence in data analysis is set to transform how we interpret experimental results. These technologies can sift through vast amounts of data generated by particle collisions, identifying patterns and anomalies that human analysts might overlook. As machine learning algorithms become more sophisticated, they will not only enhance our ability to detect new particles but also refine our theoretical models, paving the way for groundbreaking advancements in particle physics.
Q&A
-
What is the particle theory of matter?
The particle theory of matter posits that all matter is composed of tiny particles that are in constant motion. These particles can be atoms, molecules, or ions, and their behavior explains the physical properties of substances.
-
What are the four main points of the particle theory?
The four main points of the particle theory are:
- All matter is made up of particles.
- Particles are in constant motion.
- Particles have spaces between them.
- Temperature affects the speed of particles.
-
How does the particle theory explain different states of matter?
The particle theory explains that the arrangement and movement of particles determine the state of matter. In solids, particles are closely packed and vibrate in place; in liquids, they are close but can move past each other; and in gases, they are far apart and move freely.
-
Why is the particle theory important in science?
The particle theory is crucial because it provides a foundational understanding of the behavior of matter. It helps explain phenomena such as changes in state, temperature effects, and the properties of different materials, making it essential for fields like chemistry and physics.
the four particle theory offers a fascinating lens through which we can understand the fundamental building blocks of our universe. As we continue to explore these concepts, we unlock new mysteries and deepen our appreciation for the intricate dance of matter.
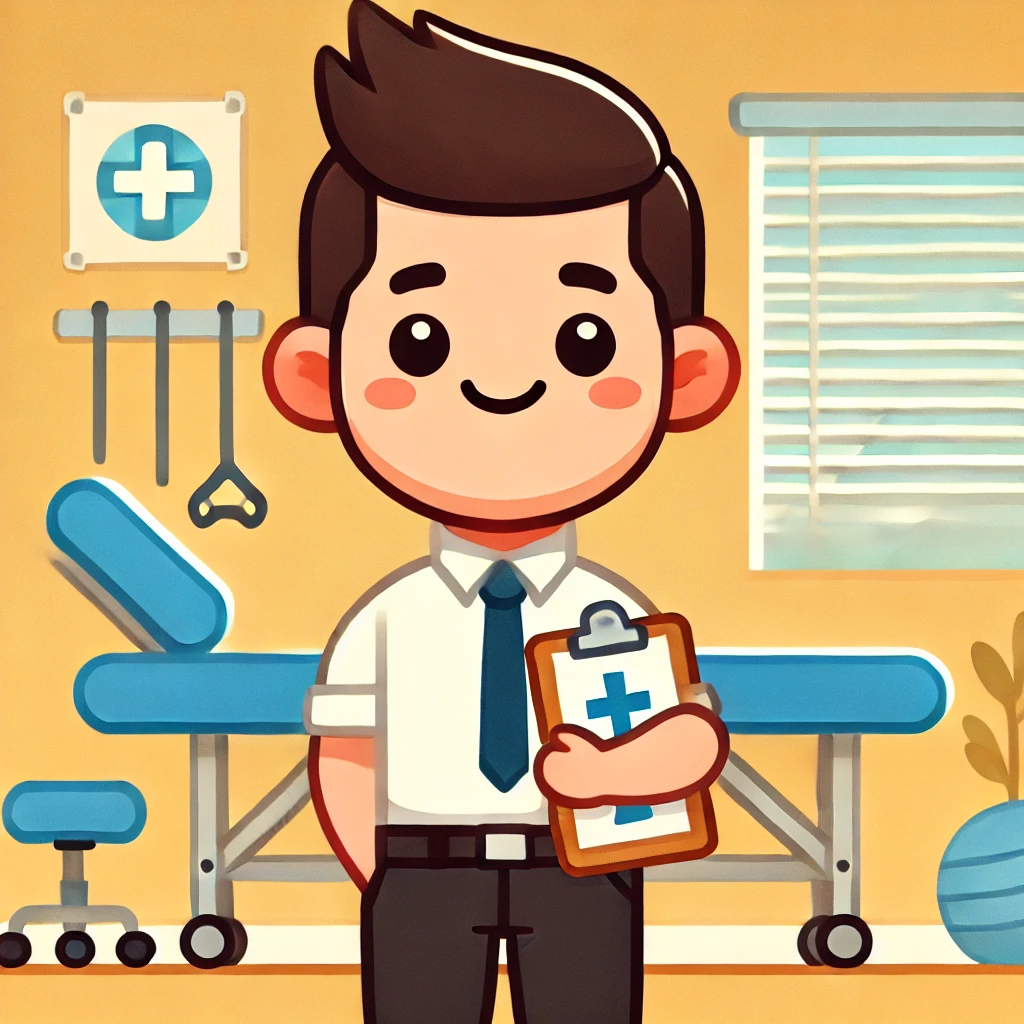
大家好,我是彼得潘,專業的手法身體治療師。我喜歡探索和研究各種主題,並透過與人工智慧的合作分享專業、實用、有趣的文章。我們定期進行人工審核,以確保內容的準確性。如果您發現文章中有任何不準確的地方,請隨時與我們聯繫,我們會及時糾正。您可以透過 [email protected] 與我們聯繫。